Announcements
VBRN Biostatistics Support in collaboration with the NNE-CTR
We welcome Jonathan Emery from the Northern New England Center for Translational Research (NNE-CTR) to VBRN! Jonathan is available to provide biostatistics support services at no charge to network faculty for their biomedical research projects. If you are interested...
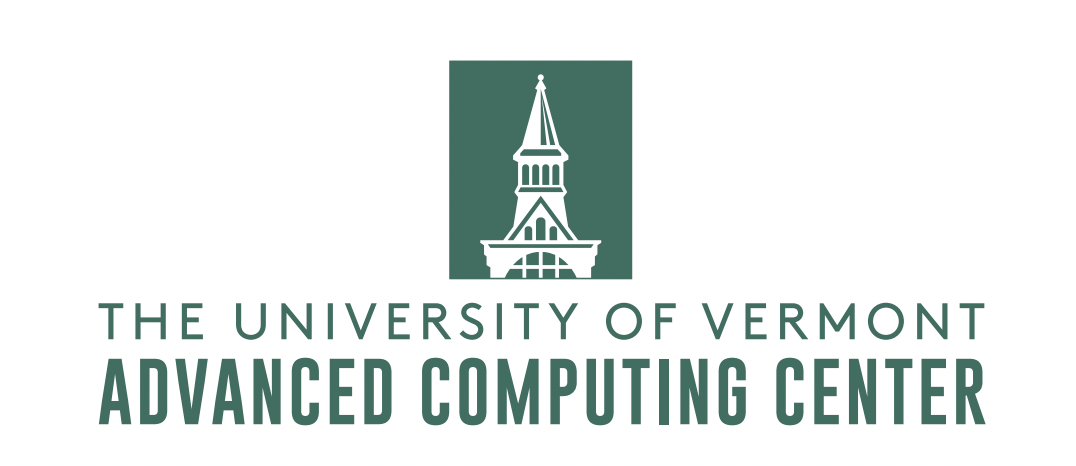
VACC-VBRN Find and Explore Program
Find and Explore is a new pilot program for partner institutions to utilize the Vermont Advanced Computing Center (VACC) at the University of Vermont. The VACC provides state-of-the-art high-performance research cyberinfrastucture, including data storage and...
VBRN Thematic Group Highlight
Bio-organic Chemistry Thematic GroupGroup Leader: Dr. Robert Hondal, Associate Professor, Department of Chemistry and Biochemistry, University of VermontMembers: Five faculty from VBRN baccalaureate partner institutions (BPIs) The Bio-organic Thematic Group provides...
Request for Information: Soliciting ideas for new NIH Common Fund programs
Request for Information: Soliciting ideas for new NIH Common Fund programsOffice of Strategic Coordination (Common Fund) The NIH Common Fund is soliciting ideas for potential new scientific programs that may be supported in fiscal year 2025 or beyond. The Common Fund...
View More